Prolonged mechanical ventilation after lung transplant: predictors and impact on survival
Highlight box
Key findings
• Prolonged mechanical ventilation (PMV) >21 days is more common in high-risk patients and complicated peri-operative course.
• Risk factors for longer weaning of mechanical ventilation included bridging on extracorporeal membrane oxygenation, duration on cardiopulmonary bypass, primary graft dysfunction (PGD) grade, re-exploration for bleeding, postoperative dialysis, and airway complications.
• Risk factors for survival were single lung transplant, postoperative dialysis, PMV >21 days, and higher PGD grade.
What is known and what is new?
• PMV >21 days is associated with worse outcomes after lung transplant.
• Long-term weaning of mechanical ventilation (>90 days) after lung transplant was associated with the worst survival rates among groups of PMV.
• Duration on cardiopulmonary bypass is associated with longer weaning of mechanical ventilation after lung transplant.
• Single lung transplant is associated with worse survival.
What is the implication, and what should change now?
• Aggressive rehabilitation during bridging to avoid PMV after lung transplant.
• Evaluation for trans-catheter therapies for cardiac pathologies prior to transplant to minimize time on pump for concomitant cardiac procedures.
• Careful selection of candidates for single lung transplant.
Introduction
Lung transplantation (LTx) represents the definitive treatment for selected candidates with end stage lung disease (1-3). However, access to LTx is substantially limited by the scarcity of transplantable donor lungs (4,5). Thus, optimal organ utilization is crucial to maximize the overall efficacy of LTx. To that end, the lung allocation score (LAS) was developed in 2005 to prioritize LTx candidates based on medical urgency rather than accrued waiting list time (6). Although LAS implementation dramatically decreased wait time and wait-list mortality, it altered the characteristics of candidates prioritized for transplant (3). Fibrotic lung disease has become the most common indication for LTx, surpassing obstructive lung disease (7). The LAS thereby prioritized older candidates who have multiple comorbidities, and/or sicker patients bridged to LTx on mechanical ventilation (MV) or extracorporeal membrane oxygenation (ECMO) (3). Not surprisingly, these recipient characteristics impact both the conduct of the LTx procedure and the postoperative course; for instance, increased use of cardiopulmonary bypass (CPB) and concomitant cardiac procedures influences the incidence of primary graft dysfunction (PGD). Given these challenges, a subgroup of LTx recipients can require a prolonged hospital course, requiring long-term weaning of MV (8-10). Recipients requiring long-term weaning of MV and intensive care unit (ICU) care are more vulnerable for complications including pneumonia, sepsis, delirium, ileus, and venous thromboembolism, which can adversely affect survival and overall benefit (9,10). In that sense, prolonged MV (PMV) itself might not be the direct cause of worse outcomes but can be used to identify this subgroup of recipients at risk. In this study, we sought to test the hypothesis that a subgroup of LTx candidates requiring PMV had less benefit from LTx.
The Centers for Medicare and Medicaid Services (CMS) considers MV >21 days an indicator of PMV (9,11-13). However, “21-day” definition of PMV in the LTx population fails to discriminate between diverse clinical courses after LTx, especially for those requiring long-term ventilator weaning courses—the focus of our study. Thus, based on our clinical observation at a specialized transplant center, we proposed to investigate four groups of MV dependence including <2 days (fast-track), 3–21 days (short weaning course), 22–90 days (long weaning course), and >90 days (very long-term weaning). We aim to identify unique baseline and peri-operative risk factors for PMV and understand the impact of PMV on survival after LTx. We present this article in accordance with the STROBE reporting checklist (available at https://ccts.amegroups.com/article/view/10.21037/ccts-23-13/rc).
Methods
Study cohort
This retrospective cohort study analyzed 1,140 LTx procedures performed at our institution from January 2008 to January 2019. Inclusion criteria were all adult lung transplant patients during the study period including single or bilateral LTx as well as multi-organ transplants. Exclusion criteria were recipients <18-year-old. Baseline demographics, perioperative variables, duration of MV, and follow-up data were collected prospectively in our transplant database and retrospectively analyzed. Chart review was used to complete any missing data of duration of MV or tracheostomy procedures. The study cohort was divided into four groups based on postoperative MV duration: group 1: ≤2 days, group 2: 3–21 days, group 3: 22–90 days, and group 4: >90 days (Table 1). In a second analysis, the duration of MV was used as a continuous variable in a logistic regression model to identify variables related to longer ventilation days, then a univariate analysis comparing the period of ventilation between different variables. The total duration of MV after LTx was calculated by adding intubation time to total time on MV, including all days with tracheostomy in place, until tracheostomy de-cannulation or discharge to a long-term acute care facility to continue MV weaning. Our perioperative management protocols include weaning of MV, prevention of ileus, and pain control. Protocol of MV weaning includes gradual expanding time off pressure support, pulmonary hygiene, and physical therapy. Primary outcome of the study was PMV >21 days and secondary outcome was survival after LTx.
Table 1
Characteristic | Non-PMV | PMV | P | |||
---|---|---|---|---|---|---|
Group 1 MV ≤2 days (n=693) | Group 2 MV 3–21 days (n=206) | Group 3 MV 22–90 days (n=185) | Group 4 MV >90 days (n=56) | |||
Female | 217 [31] | 72 [35] | 68 [37] | 21 [38] | 0.4 | |
Age at time of transplant, years | 62 [55–67] | 58 [50–63] | 59 [50–65] | 58 [45–65] | <0.001 | |
Body mass index, kg/m2 | 26 [22–29] | 27 [23–30] | 27 [22–30] | 26 [22–28] | 0.05 | |
Diabetes | 168 [24] | 66 [32] | 49 [26] | 19 [34] | 0.08 | |
Hypertension | 312 [45] | 100 [49] | 90 [49] | 27 [48] | 0.7 | |
Lung allocation score | 40 [34–52] | 47 [37–84] | 47 [37–84] | 62 [39–86] | <0.001 | |
Diagnosis | <0.001 | |||||
Obstructive lung disease | 190 [27] | 38 [18] | 37 [20] | 10 [18] | ||
Pulmonary vascular disease | 19 [3] | 12 [6] | 16 [9] | 5 [9] | ||
Suppurative lung disease | 68 [10] | 20 [10] | 14 [8] | 11 [20] | ||
Restrictive lung disease | 406 [59] | 132 [64] | 108 [58] | 26 [46] | ||
Re-transplant | 10 [1] | 4 [2] | 10 [5] | 4 [7] | ||
Bridge to transplant | ||||||
Mechanical ventilation | 230 [33] | 81 [39] | 95 [51] | 37 [66] | <0.001 | |
ECMO | 6 [1] | 14 [7] | 32 [17] | 11 [20] | <0.001 | |
Lung transplant procedure | ||||||
Double lung transplantation | 361 [52] | 159 [77] | 148 [80] | 50 [89] | <0.001 | |
Redo transplantation | 10 [1] | 4 [2] | 10 [5] | 4 [7] | 0.002 | |
CPB usage | 385 [56] | 170 [83] | 156 [84] | 51 [91] | <0.001 | |
CPB duration, minutes | 166 [128–205] | 200 [148–256] | 217 [163–278] | 241 [185–288] | <0.001 | |
Concomitant cardiac procedure | 51 [7] | 38 [18] | 23 [12] | 16 [29] | <0.001 | |
Donor | ||||||
Brain dead | 637 [92] | 186 [90] | 164 [89] | 48 [86] | 0.3 | |
DCD | 56 [8] | 20 [10] | 21 [11] | 8 [14] | 0.3 | |
Age, years | 36 [24–50] | 41 [24–52] | 42 [27–53] | 37 [24–50] | 0.05 | |
Female | 298 [44] | 99 [48] | 99 [55] | 31 [57] | 0.02 |
Data are presented as n [%] or median [IQR]. PMV, prolonged mechanical ventilation; MV, mechanical ventilation; ECMO, extracorporeal membrane oxygenation; CPB, cardiopulmonary bypass; DCD, donation after cardiac death; IQR, interquartile range.
Statistical analysis
To identify independent risk factors for PMV after LTx, multivariate analyses were performed in a stepwise logistic regression model (including covariates with a P value <0.2) using baseline characteristics and perioperative variables listed in Tables 1,2 (Appendix 1). The duration of MV in days was used as a continuous variable in the first model, then the recipients were divided into two groups based on the duration of MV and the group of recipients with PMV >21 was the target of the second model. The effect of categorical risk factor was reported as odds ratio (OR), with 95% confidence interval (CI), and its corresponding P value. Cumulative survival was determined by the Kaplan-Meier method and log-rank test was utilized for comparison among curves. Cox proportional hazard model was utilized to identify independent risk factors for survival. The effect of each risk factor on mortality was described as a hazard ratio (HR), with 95% CI, and its corresponding P value. Data analyses were performed using JMP version 15.2.0 (SAS Institute, Inc., Cary, NC, USA). Continuous variables are reported as median with interquartile range. Categorical data are summarized as units and percentages. Categorical data were compared using the chi-square test. Normally distributed continuous data were compared using the Student’s t-test and ANOVA, while Wilcoxon and Kruskal-Wallis tests were used for skewed data.
Table 2
Characteristic | Non-PMV | PMV | P | |||
---|---|---|---|---|---|---|
Group 1 MV ≤2 days (n=693) | Group 2 MV 3–21 days (n=206) | Group 3 MV 22–90 days (n=185) | Group 4 MV >90 days (n=56) | |||
ECMO after LTx | 5 [1] | 13 [6] | 24 [13] | 10 [18] | <0.001 | |
PGD grade at 72 hours | <0.001 | |||||
0 | 271 [43] | 39 [20] | 26 [14] | 2 [4] | ||
1 | 302 [48] | 85 [44] | 71 [39] | 23 [44] | ||
2 | 44 [7] | 32 [17] | 24 [13] | 9 [17] | ||
3 | 16 [2] | 36 [19] | 60 [33] | 18 [35] | ||
Re-exploration for bleeding | 28 [4] | 34 [17] | 38 [21] | 22 [39] | <0.001 | |
Dialysis initiation after LTx | 9 [1] | 18 [9] | 44 [24] | 27 [48] | <0.001 | |
Airway complication (stent) | 19 [3] | 9 [4] | 20 [11] | 9 [16] | <0.001 | |
Death during hospital stay | 6 [1] | 17 [8] | 26 [14] | 14 [25] | <0.001 | |
Acute rejection | 386 [56] | 87 [42] | 60 [32] | 14 [25] | <0.001 | |
Chronic rejection | 260 [38] | 66 [32] | 48 [26] | 11 [20] | 0.002 | |
Readmission after discharge | 650 [94] | 181 [88] | 162 [88] | 42 [75] | <0.001 |
Data are presented as n [%]. PMV, prolonged mechanical ventilation; MV, mechanical ventilation; ECMO, extracorporeal membrane oxygenation; PGD, primary graft dysfunction; LTx, lung transplantation.
Ethical consideration
The study was conducted in accordance with the Declaration of Helsinki (as revised in 2013). The study was approved by Institutional Review Board of Cleveland Clinic (IRB # 18-868) as Exempt Human Subject Research and individual consent for this retrospective analysis was waived.
Results
Univariate (unmatched) analysis of duration of MV after LTx
The overall incidence of PMV >21 days in the whole cohort was 21% (n=241), including 16% with MV 22–90 days and 5% with MV >90 days. 61% had MV ≤2 days and 18% required MV 3–21 days after LTx (Table 1). Between the four groups, there was a significant difference in recipient age (P<0.001) and LAS (highest in group 4, P<0.001), Figure 1A. Restrictive lung disease was the most common indication for LTx followed by obstructive lung disease (P<0.001). Bridging on MV and ECMO before LTx was highest in group 4 (P<0.001). In terms of LTx procedures, group 4 (PMV >90 days) had the highest rates of bilateral LTx (P<0.001), redo LTx (P=0.002), concomitant cardiac procedures (P<0.001), and CPB usage and duration (P<0.001), Figure 1B. On the donor side, there were no significant differences in the donor type (DCD vs. brain dead), age, or gender among the groups (Table 1).
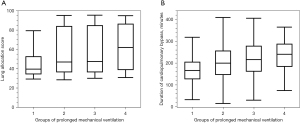
Postoperatively, group 3 and group 4 (PMV >21 days) had significantly higher ranges of PGD grade 3 at 72 hours, need for ECMO insertion, re-exploration for bleeding, initiation of renal replacement therapy, and airway complication requiring stent (Table 2, P<0.001). Rates of acute and chronic rejection were lower in group 3 and group 4. There was a significant difference in early mortality among the groups; highest rates of in-hospital mortality occurred in groups 3 and 4 (PMV >21 days), P<0.001.
When duration of MV was used as a continuous variable in the analysis model (Table 3), factors associated with longer duration of MV were redo LTx, bridging to LTx (ECMO and MV), double LTx, usage of CPB, concomitant cardiac procedure, post-LTx ECMO, higher grades of PGD, re-exploration for bleeding, post-LTx dialysis, and airway complications requiring stent (P<0.001). Longer CPB duration (Spearman’s ρ=0.31, P<0.001), higher recipient body mass index (Spearman’s ρ=0.08, P=0.009), younger recipient age (Spearman’s ρ=−0.19, P<0.001), higher LAS (Spearman’s ρ=0.25, P<0.001), and older donor age (Spearman’s ρ=0.61, P=0.04) were associated with longer duration of MV after LTx.
Table 3
Variable | Median days on mechanical ventilation [IQR] | P |
---|---|---|
Diagnosis | <0.001 | |
Obstructive lung disease | 1 [1–4] | |
Pulmonary vascular disease | 4 [2–58.5] | |
Suppurative lung disease | 2 [1–15] | |
Restrictive lung disease | 2 [1–9] | |
Re-transplant | 13.5 [1.25–58.75] | |
Bridge to transplant | ||
Mechanical ventilation | <0.001 | |
Yes | 2 [1–27] | |
No | 1 [1–4] | |
ECMO | <0.001 | |
Yes | 31 [14–60] | |
No | 1 [1–6] | |
Donor | ||
Type | 0.01 | |
Brain dead | 2 [1–8] | |
DCD | 2 [1–24] | |
Gender | 0.02 | |
Male | 1.5 [1–5] | |
Female | 2 [1–21] | |
Lung transplant procedure | ||
Double LTx | 2 [1–26] | <0.001 |
Single LTx | 1 [1–2] | |
Primary LTx | 2 [1–9] | 0.001 |
Redo LTx | 13.5 [1.25–58.75] | |
CPB | <0.001 | |
Yes | 2 [1–25] | |
No | 1 [1–2] | |
Concomitant cardiac procedure | <0.001 | |
Yes | 4 [1–37.25] | |
No | 1 [1–7] | |
ECMO placed after LTx | <0.001 | |
Yes | 30.5 [12.5–59.5] | |
No | 1 [1–6] | |
PGD grade at 72 hours | <0.001 | |
0 | 1 [1–2] | |
1 | 2 [1–6] | |
2 | 4 [1–29.5] | |
3 | 27.5 [5.75–60.25] | |
Re-exploration for bleeding | <0.001 | |
Yes | 3 [1–18] | |
No | 1 [1–5] | |
Dialysis initiation after LTx | <0.001 | |
Yes | 42.5 [17.75–115.25] | |
No | 1 [1–4] | |
Airway complication (stent) | <0.001 | |
Yes | 24 [2–56.5] | |
No | 2 [1–7] |
IQR, interquartile ratio; ECMO, extracorporeal membrane oxygenation; LTx, lung transplantation; CPB, cardiopulmonary bypass.
Predictors of PMV after LTx
We first examined the independent risk factors for longer MV after LTx which included initiation of renal replacement therapy postoperative (hemodialysis, P<0.001), PGD grade (P=0.001), airway complication requiring stent (P=0.001), re-exploration for bleeding (P=0.004), bridging on MV (P=0.005), duration of CPB during LTx (P=0.01), acute rejection (P=0.01), and bridging on ECMO (P=0.02).
Next, we examined independent risk factors specifically for PMV >21 days. and we found similarly that PGD grade (P<0.001), initiation of renal replacement therapy postoperative (hemodialysis, OR 5.71, 95% CI: 3.2–10.2, P<0.001), bridging on ECMO (OR 5.53, 95% CI: 2.7–11.1, P<0.001), Double LTx (OR 2.61, 95% CI: 1.7–3.9, P<0.001), airway complication requiring stent (OR 3.79, 95% CI: 1.9–7.3, P<0.001), and re-exploration for bleeding (OR 1.97, 95% CI: 1.2–3.3, P=0.01) were significant predictors for PMV >21 days after LTx.
Survival
Overall survival rates in the study cohort were 85.9%, 67.5%, and 53.8 at 1, 3, and 5 years, respectively (Figure 2). There was a significant difference in survival among the four groups with lowest survival rates in group 4 (PMV >90 days), P<0.001 (Figure 3). Independent risk factors for worse survival after LTx in this cohort were post-operative initiation of renal replacement therapy (hemodialysis HR 2.65, 95% CI: 1.9–3.6, P<0.001), single LTx (HR 1.98, 95% CI: 1.6–2.4, P<0.001), recipient hypertension (HR 1.22, 95% CI: 1.1–1.4, P=0.002), PMV >21 days after LTx (HR 2.18, 95% CI: 1.7–2.8, P=0.003), and higher PGD grade after LTx (P=0.03).
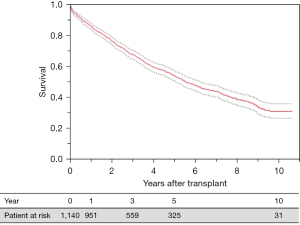
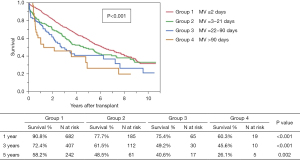
Discussion
The LAS system was instituted in 2005 to ensure optimal organ utilization, prioritizing candidates based on medical urgency (6). Reviewing our LTx program’s cumulative experience over the last three decades confirms that LAS implementation resulted in older recipient age, fibrotic lung disease as the leading LTx indication, and a greater burden of recipient comorbidities reflected in increased concomitant cardiac procedures and CPB usage (3). In the same study, high pre-LTx LAS was associated with severe PGD and early in-hospital mortality after LTx. Clinically, we observed that those LTx recipients requiring long-term MV support become vulnerable to multiple complications and worse survival. Therefore, we sought to test the hypothesis that a subgroup of LTx candidates requiring long-term MV had less benefit from LTx. Consequently, in this study our aims included (I) to define the characteristics of LTx recipients who required PMV after LTx, (II) to identify the predictors of PMV, and (III) to quantitate the impact of PMV on survival.
The fate of long-term weaning of MV after LTx
Patients requiring PMV >21 days after LTx had significantly higher in-hospital mortality and lower overall survival, consistent with the multivariate analysis showing PMV >21 is s an independent risk factor for mortality after LTx. Previously, Hadem et al. also reported increased in-hospital death and worse survival in patients who required PMV >21 days (cohort 2005–2012; PMV incidence 14%); however, conditional long-term outcomes were not different among patients surviving to hospital discharge (9). In their analysis of 690 LTx recipients, PMV >21 days was not a predictor of mortality. Another measure of prolonged course after LTx is length of stay (LOS). In an analysis of the United Network of Organ Sharing (UNOS) database (n=12,647; 2006–2014), Banga et al. reported LOS >25 days (incidence 26%) independently predicted mortality at 1 and 5 years after LTx (14). Huddleston et al. also showed that tracheostomy within 30 days of LTx (2005–2016: incidence 10%) was associated with worse 1- and 3-year survival (10). While previous evidence has shown that long-term weaning of MV after LTx negatively impacts survival, our analysis demonstrates that longest term weaning group (group 4, >90 days) had remarkably worse survival.
Characteristics of long-term weaning of MV after LTx
Univariate analysis based on duration of MV after LTx identified baseline and peri-operative characteristics associated with PMV. These included higher LAS score and bridging to LTx on MV and/or ECMO. The LTx procedures were notable for more concomitant cardiac procedures and increased/longer CPB use during LTx. Early after surgery, these patients had a higher incidence of severe PGD, more frequent need for ECMO placement after LTx, re-exploration for bleeding, initiation of hemodialysis, and stenting for airway complications. The majority of these characteristics have also correlated with PMV in other studies after LAS implementation (9,10), as well as in the UNOS study for patients with prolonged LOS >25 days (14). In short, a consequence of transplanting sicker patients with higher LAS scores appears to be increased risk of PMV, although wait-list mortality is decreasing. Apart from identification of patients at risk for PMV at the time of listing, development of additional strategies directed at modifying the risk of PMV after LTx continue to be needed. In the recent years of the study, we dedicated an intensive care unit for lung transplant patients that is managed by a consistent team of intensivists and respiratory therapists to facilitate the process of MV weaning. Our approach is early tracheostomy after transplant if the patient is not making progress on extubation trials for few days, or failed extubation leading to re-intubation, to facilitate weaning of MV and physical therapy.
Predictors of long-term weaning of MV after LTx
The room for modifications varies among risk factors for PMV. Bridging on ECMO, PGD, or dialysis can be unavoidable while listing for single LTx or concomitant cardiac procedures may have more room for alterations. Candidates bridged to LTx with MV and/or ECMO are at higher risk for long-term weaning from MV postoperatively. Hadem et al. have shown that pre-LTx MV was a predictor of PMV >21 days, but the study did not specifically analyze subgroups of patients who required MV for longer periods (9). In the UNOS data analysis, Banga et al., reported bridging to LTx with MV or ECMO was a predictor for prolonged LOS >25 days after LTx (14). This could reflect limited rehabilitation of candidates being bridged on MV alone or placed on ECMO after a period of deconditioning while on high oxygen requirement. Thus, early ECMO placement for bridging is advisable in appropriately selected candidates, especially single site-dual cannula, to allow more aggressive rehabilitation by avoiding or as an adjunct to MV (15,16).
In our analysis, the two most important risk factors for PMV were high PGD grade and dialysis, which also predicted lower survival. Undoubtedly, high grade PGD remains a significant challenge for LTx early outcomes (3,17). Huddleston et al. reported the association between PGD grade 3 and tracheostomy or death within 30 days after LTx (10). Recently, two perioperative strategies have shown promise in reducing or preventing high grade PGD. Use of intraoperative veno-arterial (VA) ECMO permits controlled donor lung reperfusion and stabilized intra-procedural hemodynamics, while avoiding side effects associated with longer CPB duration (18-20). In addition, utilization of ex-vivo lung perfusion (EVLP) for evaluation of marginal donor lungs has improved donor lung selection and continues to show promise for modifying their function (21,22).
Postoperative dialysis complicates weaning from MV due to multiple factors, including ensuring that adequate volume removal required to facilitate weaning of MV is tolerated hemodynamically. Dialysis also limits patient mobility and rehabilitation in the postop period. From previous studies, known risk factors for postoperative dialysis post-LTx include lower pre-LTx creatinine clearance, right ventricular dysfunction, peri-operative bleeding, and hemodynamic instability, and exposure to calcineurin inhibitors (23-25). It is critical to identify recipients at risk of acute renal dysfunction after LTx and tailor their immunosuppression plan to limit exposure to nephrotoxic agents in the early phase after surgery. Optimizing surgical techniques is to continue to avoid blood loss, transfusion, and periods of hemodynamic instability.
In our analysis, single LTx was not a risk factor for PMV >21 days, but a predictor of worse survival after LTx. Similarly, Huddleston et al. reported that single LTx was not a risk factor for tracheostomy within 30 days after LTx (10). At our center, we consider single LTx mainly for candidates with favorable lung perfusion split, no significant pulmonary hypertension, and chronologically older candidates with some frailty. The rationale is to reduce the magnitude of the surgery in favor of increasing the possibility of a faster recovery. However, as shown in this study analysis, this strategy may carry risk for a prolonged post-LTx course, particularly if the recipient develops complications in the single allograft such as PGD, infection, or rejection. A prolonged postoperative course in this population may contribute to lower survival rates for single LTx vs. bilateral LTx (3,26). Schaffer et al. also reported lower graft survival in patients with fibrotic lung disease who underwent single LTx compared to bilateral LTx, while observing no survival difference in single vs. bilateral LTx in patients with obstructive lung disease (27). In LTx candidates who may have limited physical reserve to tolerate bilateral LTx, specialized rehabilitation or other measures to mitigate frailty may be required to ensure that the patient is a reasonable LTx candidate. Other measures for single LTx can be matching to ideal donor, limit cold ischemia time, strict precautions for aspiration, and early aggressive intervention for ileus or infection.
Other important predictors for PMV >21 days were (I) duration of CPB during LTx and (II) re-exploration for bleeding after LTx, which are consistent with the observed increased rate of concomitant cardiac procedures in recipients with PMV >21 days. In the current era of rapidly evolving trans-catheter therapies for coronary and valvular pathology, addressing any coexisting cardiac pathology, when feasible before LTx listing such as trans-catheter aortic valve replacement (28,29) and percutaneous coronary intervention (30,31) deserves consideration when patients are considered for LTx listing.
Limitations
Although this is a cohort of over 1,000 cases with prospectively collected data, it is a single-center retrospective analysis and limited by its observational nature which is subject to internal bias. All days with tracheostomy in place (used intermittently or capped) was included in the MV duration to avoid variability. Length of weaning of MV after discharge to long term acute care facilities were not available for out of state facilities or those not affiliated with our institution. A complete set of granular data accounting for pain control, which would be largely subjective, and ileus were not available to include in this analysis. Pain control and ileus could be factors that influence early weaning of MV but likely not ongoing long-term weaning, especially with standardized postoperative protocols such as epidural catheters and bowel regimens. The UNOS database does not specifically record data on very long-term weaning of MV after LTx, which limited our ability to conduct a national sample analysis.
Conclusions
We undertook this study to better understand which LTx candidates have increased risk of PMV, and protracted ICU course which limit the benefit of LTx. We identified specific LTx recipient characteristics within subgroups of PMV, including recipients who had very prolonged vent weaning (>90 days). Efforts to continue early rehabilitation for bridging-to-LTx, reducing the incidence of PGD, and dialysis, however these factors could be less modifiable. Areas for potential improvement is careful selection of candidates for single LTx and trans-catheter therapies for co-existing cardiac pathology when feasible. Our single center study’s findings provide a basis for future broader studies aimed at avoiding PMV in LTx recipients.
Acknowledgments
Our abstract has been accepted for presentation at the ISHLT meeting 2022, be held 27−30 April 2022 in Boston, Massachusetts.
Funding: None.
Footnote
Reporting Checklist: The authors have completed the STROBE reporting checklist. Available at https://ccts.amegroups.com/article/view/10.21037/ccts-23-13/rc
Data Sharing Statement: Available at https://ccts.amegroups.com/article/view/10.21037/ccts-23-13/dss
Peer Review File: Available at https://ccts.amegroups.com/article/view/10.21037/ccts-23-13/prf
Conflicts of Interest: All authors have completed the ICMJE uniform disclosure form available at https://ccts.amegroups.com/article/view/10.21037/ccts-23-13/coif). K.R.M. is a consultant to Lung Bioengineering and Transmedics. H.E. has financial relationship with Edwards Lifesciences & LifeNet Health. The other authors have no conflicts of interest to declare.
Ethical Statement: The authors are accountable for all aspects of the work in ensuring that questions related to the accuracy or integrity of any part of the work are appropriately investigated and resolved. The study was conducted in accordance with the Declaration of Helsinki (as revised in 2013). The study was approved by Institutional Review Board of Cleveland Clinic (IRB # 18-868) as Exempt Human Subject Research and individual consent for this retrospective analysis was waived.
Open Access Statement: This is an Open Access article distributed in accordance with the Creative Commons Attribution-NonCommercial-NoDerivs 4.0 International License (CC BY-NC-ND 4.0), which permits the non-commercial replication and distribution of the article with the strict proviso that no changes or edits are made and the original work is properly cited (including links to both the formal publication through the relevant DOI and the license). See: https://creativecommons.org/licenses/by-nc-nd/4.0/.
References
- Hartert M, Senbaklavacin O, Gohrbandt B, et al. Lung transplantation: a treatment option in end-stage lung disease. Dtsch Arztebl Int 2014;111:107-16. [PubMed]
- Kugler C, Gottlieb J, Warnecke G, et al. Health-related quality of life after solid organ transplantation: a prospective, multiorgan cohort study. Transplantation 2013;96:316-23. [Crossref] [PubMed]
- Elgharably H, Ayyat KS, Okamoto T, et al. Evolution of Recipient Characteristics Over 3 Decades and Impact on Survival After Lung Transplantation. Transplantation 2021;105:e387-94. [Crossref] [PubMed]
- Yeung JC, Keshavjee S. Overview of clinical lung transplantation. Cold Spring Harb Perspect Med 2014;4:a015628. [Crossref] [PubMed]
- Elgharably H, Shafii AE, Mason DP. Expanding the donor pool: donation after cardiac death. Thorac Surg Clin 2015;25:35-46. [Crossref] [PubMed]
- Christie JD, Carby M, Bag R, et al. Report of the ISHLT Working Group on Primary Lung Graft Dysfunction part II: definition. A consensus statement of the International Society for Heart and Lung Transplantation. J Heart Lung Transplant 2005;24:1454-9. [Crossref] [PubMed]
- Egan TM, Edwards LB. Effect of the lung allocation score on lung transplantation in the United States. J Heart Lung Transplant 2016;35:433-9. [Crossref] [PubMed]
- Olsen JE, Auråen H, Durheim MT, et al. Predictors of long intensive care need after lung transplantation. Clin Transplant 2021;35:e14152. [Crossref] [PubMed]
- Hadem J, Gottlieb J, Seifert D, et al. Prolonged Mechanical Ventilation After Lung Transplantation-A Single-Center Study. Am J Transplant 2016;16:1579-87. [Crossref] [PubMed]
- Huddleston SJ, Brown R, Rudser K, et al. Need for tracheostomy after lung transplant predicts decreased mid- and long-term survival. Clin Transplant 2020;34:e13766. [Crossref] [PubMed]
- MacIntyre NR, Epstein SK, Carson S, et al. Management of patients requiring prolonged mechanical ventilation: report of a NAMDRC consensus conference. Chest 2005;128:3937-54. [Crossref] [PubMed]
- Gomez C, Doddoli C, Coltey B, et al. Prolonged mechanical ventilation negatively affects survival and functional outcome in lung transplant recipients. Eur Respir J 2015;46:PA4550.
- Gomez C, Doddoli C, Coltey B, et al. Prolonged Mechanical Ventilation in Lung Transplant Recipients: Predictive Factors and Outcomes. J Heart Lung Transplant 2013;32:S270. [Crossref]
- Banga A, Mohanka M, Mullins J, et al. Hospital length of stay after lung transplantation: Independent predictors and association with early and late survival. J Heart Lung Transplant 2017;36:289-96. [Crossref] [PubMed]
- Chiumello D, Coppola S, Froio S, et al. Extracorporeal life support as bridge to lung transplantation: a systematic review. Crit Care 2015;19:19. [Crossref] [PubMed]
- Lee SH. Awakening in extracorporeal membrane oxygenation as a bridge to lung transplantation. Acute Crit Care 2022;37:26-34. [Crossref] [PubMed]
- Christie JD, Kotloff RM, Ahya VN, et al. The effect of primary graft dysfunction on survival after lung transplantation. Am J Respir Crit Care Med 2005;171:1312-6. [Crossref] [PubMed]
- Ayyat KS, Elgharably H, Okamoto T, et al. Lung Transplantation on Cardiopulmonary Bypass: Time Matters. J Heart Lung Transplant 2020;39:S65. [Crossref]
- Weingarten N, Schraufnagel D, Plitt G, et al. Comparison of mechanical cardiopulmonary support strategies during lung transplantation. Expert Rev Med Devices 2020;17:1075-93. [Crossref] [PubMed]
- Elgharably H, Javorski MJ, McCurry KR. Bilateral sequential lung transplantation: technical aspects. J Thorac Dis 2021;13:6564-75. [Crossref] [PubMed]
- Ayyat KS, Okamoto T, Niikawa H, et al. A CLUE for better assessment of donor lungs: Novel technique in clinical ex vivo lung perfusion. J Heart Lung Transplant 2020;39:1220-7. [Crossref] [PubMed]
- Cypel M, Yeung JC, Liu M, et al. Normothermic ex vivo lung perfusion in clinical lung transplantation. N Engl J Med 2011;364:1431-40. [Crossref] [PubMed]
- Mason DP, Solovera-Rozas M, Feng J, et al. Dialysis after lung transplantation: prevalence, risk factors and outcome. J Heart Lung Transplant 2007;26:1155-62. [Crossref] [PubMed]
- Jing L, Chen W, Guo L, et al. Acute kidney injury after lung transplantation: a narrative review. Ann Transl Med 2021;9:717. [Crossref] [PubMed]
- Lertjitbanjong P, Thongprayoon C, Cheungpasitporn W, et al. Acute Kidney Injury after Lung Transplantation: A Systematic Review and Meta-Analysis. J Clin Med 2019;8:1713. [Crossref] [PubMed]
- Chambers DC, Yusen RD, Cherikh WS, et al. The Registry of the International Society for Heart and Lung Transplantation: Thirty-fourth Adult Lung And Heart-Lung Transplantation Report-2017; Focus Theme: Allograft ischemic time. J Heart Lung Transplant 2017;36:1047-59. [Crossref] [PubMed]
- Schaffer JM, Singh SK, Reitz BA, et al. Single- vs double-lung transplantation in patients with chronic obstructive pulmonary disease and idiopathic pulmonary fibrosis since the implementation of lung allocation based on medical need. JAMA 2015;313:936-48. [Crossref] [PubMed]
- Wallen TJ, Arnaoutakis GJ, Beaver T, et al. Successful bridge to lung transplantation with transcatheter aortic valve replacement. Am J Transplant 2020;20:3658-61. [Crossref] [PubMed]
- Chahine J, Gajulapalli RD, Kadri AN, et al. Outcomes of Transcatheter Aortic Valve Replacement in Transplant Recipients. Structural Heart 2020;4:329-33. [Crossref]
- Castleberry AW, Martin JT, Osho AA, et al. Coronary revascularization in lung transplant recipients with concomitant coronary artery disease. Am J Transplant 2013;13:2978-88. [Crossref] [PubMed]
- Koprivanac M, Budev MM, Yun JJ, et al. How important is coronary artery disease when considering lung transplant candidates? J Heart Lung Transplant 2016;35:1453-61. [Crossref] [PubMed]
Cite this article as: Hu L, Ayyat KS, Weingarten N, Okamoto T, Lehr C, Budev MM, Ahmad U, Yun JJ, McCurry KR, Elgharably H. Prolonged mechanical ventilation after lung transplant: predictors and impact on survival. Curr Chall Thorac Surg 2024;6:4.